Corrosion of reinforced concrete Corrosion – Application Note 22
Latest updated: July 26, 2023Abstract
Reinforced concrete is the most widely used composite material for building construction. The service life of this material depends on the corrosion of the steel reinforcement in concrete mainly due to the chloride (Cl–) and dioxide species (CO2). One method to protect and reduce the corrosion rate of the reinforced concrete is called electrochemical realkalization.
In this note, the corrosion process of a metallic rod in a concrete block was investigated. The realkalinization of the sample was performed in alkaline solution using chronopotentiometric technique. The effect of the realkalization on the corrosion rate was estimated using Impedance, Linear polarization and cyclic potentiodynamic polarization
Introduction
During its lifetime, the metallic structure of a building is attacked by the environment, especially by CO2. Indeed, the iron is stable in basic media inside the concrete (pH = 12-14), but carbonates, dissolved in water, migrate through the concrete to the metallic sub-structure. This phenomenon implies a decrease of the pH localized around the metallic structure. This pH shift is represented by an arrow in the Pourbaix diagram [1] in Figure 1. Consequently, at this acidic pH, the iron is no longer passivebut is actively corroding. This means that the strength of the building is affected. For instance, the “Tour Perret” in Grenoble (Figure 2), which is the first building to be made of reinforced concrete in Europe in 1924, is currently falling apart because of this process.
Figure 1: Pourbaix diagram of iron [1].
In this context, an electrochemical process was developed to keep the vicinity of the metallic rods used in reinforced concrete in basic conditions [2-7]. In order to achieve this, the metal to preserve, which in this experiment is a cathode, is immersed into a basic electrolytic paste (K2CO3 or Na2CO3) with an electrode inserted into it (Figure 3). The reduction of the water carried out through electrolysis at the electrode in the basic media produces OH–. Hydroxide ions migrate to the reinforced concrete, creating a basic environment around the metallic rod (Eq. 1).
$$H_2O+e^-\rightarrow\mathrm{\mathrm{H}}\mathrm{O}^-+\frac{1}{2}H_2 \tag{1}$$
Figure 2: The first building to be made of reinforced concrete: the “Tour Perret” in Grenoble.
Figure 3: Scheme of realkalization method.
In this note, the corrosion process of the metallic rod in a concrete block is investigated. Realkalization (electrolysis in basic media) is performed, and the benefits of the treatment are checked by Cyclic Potentiodynamic Polarization (CPP).
Experimental Conditions
Investigations are carried out with the VMP3 instrument driven by EC-Lab® software in NaCl (3%) or NaOH solution (0.4 mol·L-1). The concrete block is immersed for two days into the solution before the measurements take place.
A three-electrode set-up is used:
- Steel rod inside the concrete block as a working electrode with a surface area: A = 10 cm2 (Figure 4),
- Ag/AgCl electrode as reference electrode,
- Alloy wire as the counter electrode.
Figure 4: Scheme of concrete reinforced block.
Results
Calculation of Polarization Resistance
First of all, we have to check if the ohmic drop (RΩ) is negligible compared to the polarization resistance (Rp) of the system such that Tafel relatioships can be applied [8,9]. These two characteristics can be determined by electrochemical impedance spectroscopy (EIS) measurement (Figure 5). RΩ = 202 Ω is negligible versus Rp > 12,800 Ω (Fig. 6). Consequently, the conditions of “Tafel Fit” are respected.
Rp determination is also possible using voltamperometric measurements under a steady-state condition (very slow scan rate, i.e. 2.5 mV·min-1) and in a narrow potential range (± 10 mV around the Open Circuit Voltage, i.e. -547 mV vs. Ag/AgCl). The voltamperogramm and the “Rp Fit” are displayed in Figure 7 and give Rp = 11,744 Ω.
Figure 5: “Parameters Settings” window of potentiostatic electrochemical impedance spectroscopy measurements (PEIS) performed in NaCl (3%).
Figure 6: Nyquist diagram of concrete block.
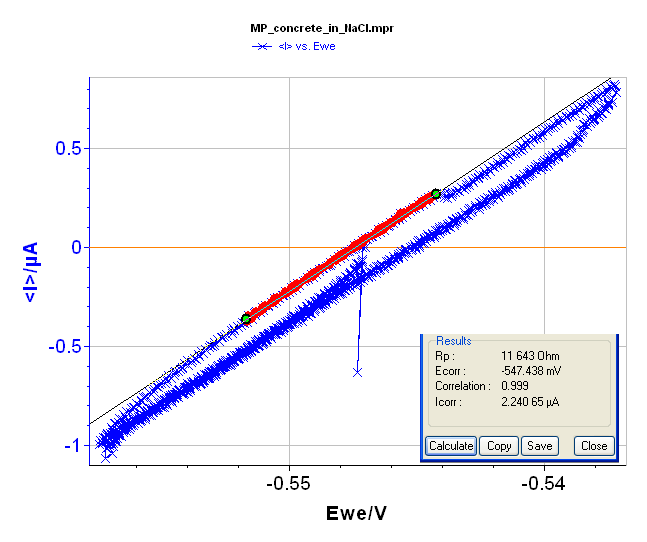
Figure 7: Voltamperogramm and “Rp Fit”of the block in NaCl (3%).
Realkalization
Realkalization treatment is performed with the chronopotentiometric (CP) techniques in NaOH (0.4 mol·L-1; pH = 13) for 66 h (Figure 8) at Is = -10 mA. Potential and charge during the electrolysis are plotted in Figure 9. At the end of the electrolysis, a stable potential of -2.4 V vs. Ag/AgCl is reached.
Figure 8: “Parameters Settings” Window of chronopotentiometry (CP).
Figure 9: Plot of potential (blue curve) and charge (red curve) during the electrolysis.
CHARACTERIZATIONS OF THE METALLIC ROD.
In order to check the efficiency of the realkalization, CPP experiments are carried out before and after the treatment. The parameters of these experiments are given in Figure 10.
Figure 10: “Parameters Settings” Window of CPP experiments.
A comparison between CPP before and after realkalization (Figure 11) displays a cathodic shift due to steel rod reduction. “Tafel Fit” analysis is performed for both curves and gives Ecorr = -616 and -1077 mV vs. Ag/AgCl for the CPP measurement performed before and after treatment, respectively.
Other parameters (Icorr, βc, βa, and corrosion rate) are computed (Figure 11 and Table ). The corrosion rate is given by Eq. 2:
$$CR=I_{corr}K\frac{EW}{\left(d\cdot A\right)} \tag{2}$$
in which K is a constant, EW is the equivalent weight, d is the density, and A is the surface area of the electrode. In the case of steel, EW and d are 18.616 g/eq. and 7.8 g·cm-3, respectively.
These fits show that corrosion rate is 50% higher before than after realkalization. This decrease of the corrosion rate demonstrates the efficiency of the realkalization process.
Figure 11: Evans diagram of the reinforced concrete before (in red) and after (in blue) realkalization (top) and “Tafel Fit” results (bottom).
Table I: Data from CPP investigations.
Before | After | |
---|---|---|
Ecorr/mV vs. Ag/AgCl | -616 | -1097 |
Icorr/µA | 404 | 272 |
βc/mV vs. Ag/AgCl | 646 | 240 |
βa/mV vs. Ag/AgCl | 670 | 325 |
Corrosion rate/mmpy* | 0.315 | 0.213 |
*mmpy: mm per year
Conclusion
This note demonstrates that electrochemical techniques are able to recondition (electrolysis) and characterize (CPP, impedance, and their corresponding analysis) the metallic structure of buildings.
It is a good example of the contribution of electrochemistry to the field of civil engineering.
Data files can be found in :
C:\Users\xxx\Documents\EC-Lab\Data\Samples\Corrosion\ PEIS_concrete_in_NaCl, MP_concrete_in_NaCl, CP_Realkalisation_NaOH, CPP_Before_Realkalisation and CPP_After_Realkalisation
References
- M. Pourbaix, in : Gauthier-Villars (Ed.) Atlas d’équilibre électrochimiques, Paris (1963).
- E. Cailleux, E. Marie-Victoire, in : L’actualité chimique, n°312-313 (2007) 22.
- http://www.novbeton.com/html/index5.html
- N. Davison, G. Glass, A. Roberts, Transportation Research Board, 87th Annual Meeting (2008).
- D. A. Koleva, K. van Breugel, J. H. W. de Wit, E. van Westing, N. Boshkov, A. L. A. Fraaij, J. Electrochem. Soc., 154 (2007) E45.
- D. A. Koleva, J. H. W. de Wit, K. van Breugel, Z. F. Lodhi, E. van Westing, J. Electrochem. Soc., 154 (2007) P52.
- D. A. Koleva, J. H. W. de Wit, K. van Breugel, Z. F. Lodhi, G. Ye, J. Electrochem. Soc., 154 (2007), C261.
- M. Stern, A. L. Geary, J. Electrochem. Soc., 104 (1957) 56.
- D. Landolt, Traité des Matériaux, Vol. 12, Presses Polytechniques et Universitaires Romandes, Lausanne (2003).
Revised in 08/2019